RESEARCH
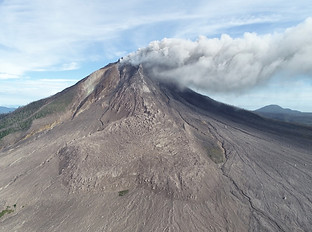
Sinabung Volcano, Indonesia
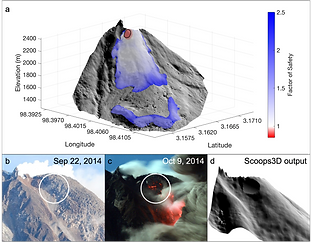
Above: Comparison of collapse hazard produced by a slope stability model to an observed collapse at Sinabung, from Carr et al, 2022, Frontiers in Earth Science. Below: Lava thickness map and flow cross sections for the Sinabung lava flow produced from SfM photogrammetry, from Carr et al., 2019, JVGR.

The Stability of Viscous Lavas:
Understanding the Driving Processes and Greatest Hazards
I am addressing the processes controlling the stability of high-viscosity, high-silica lava extruded during an effusive eruption. Silicic effusion eruptions are long-lived and present a persistent hazard to the populations living around them. The primary hazard of these eruptions is the development and collapse of instabilities in the erupted lava that form dangerous block-and-ash pyroclastic flows, plumes, and ash clouds. Dome collapse can also trigger explosive eruption phases. Developing instabilities can be difficult to observe and their specific causes at individual eruptions are not well understood. As a result, it is difficult to assess the risk of a potential collapse.
I use numerical modeling and observations to investigate the driving processes of dome collapse. Using UAS, photogrammetry, and a slope stability model, I assessed lava dome collapse hazards at Sinabung Volcano, Indonesia. Combined with a dense observational record of this multi-year eruption derived from satellites and data from Indonesian collaborators, I demonstrated that the risk of collapse persisted during periods of relatively low activity and eruptive pauses, when the hazard might otherwise be considered to have decreased.
Viscous lava can also form lava flows, which modify the collapse hazard. From January-October 2014, a 3km long andesite lava flow was emplaced at Sinabung. I conducted field campaigns in 2014 and 2018 to better understand the driving mechanisms of flow emplacement through a combination of photogrammetry and satellite remote sensing. I showed that a decrease in flow advance rate was associated with a change in the controlling mechanism of advance (from crust yield strength to core yield strength) and not a decrease in effusion rate. To accommodate the steadily erupting lava, the flow inflated to over 100 meters thick in places. Inflation of the flow led to the development of topography-controlled instabilities, which would later collapse, leading to a period of increased collapse activity and hazard, despite a decreasing eruption rate. I was also able to estimate the volume of the lava flow at 0.1 cubic kilometer, which is a long-term average eruption rate of 4.8 cubic meters per second for 2014. By 2018, the total erupted volume had increased to 0.16 cubic kilometers, a rate of 0.5 cubic meters per second since 2014.
Lava Flow Emplacement Hazards and Dynamics
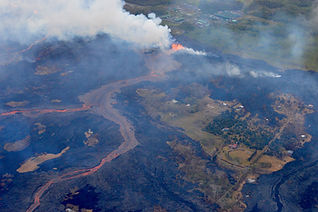
Above: Fissure 22 erupts and feeds a lava flow in Kilauea's LERZ in May 2018.
Below: Halemaʻumaʻu crater at the summit of Kīlauea in September 2021 on the first day of the 2nd of now 5 summit eruptions since 2018.
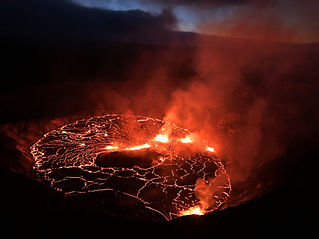
Recent well-observed eruptions of basaltic volcanoes such as Kīlauea Volcano on the Big Island of Hawaiʻi and Fagradalsfjall in Iceland offer the opportunity to study volcanic processes such as eruptive cycles and lava flow emplacement that are broadly applicable to other less-active or accessible- but still hazardous- volcanoes around the world. The proximity of these eruptions to human infrastructure also highlights the hazards posed by these persistently active volcanic systems, and a goal of my work is to improve hazard assessment through a better understanding of eruption process.
As a Mendenhall Postdoctoral Fellow with the US Geological Survey, I had the opportunity to participate in monitoring and research at Kīlauea as a staff member at the Hawaiian Volcano Observatory. This led to two ongoing research projects motivated by Kīlauea's recent eruptions.
-
I am using Kīlauea's 2018 Lower East Rift Zone eruption as a case study to better understand the controls of lava flow advance rate and the impact of syn-eruptive lava emplacement and small-scale topographic features on flow path. My goal is to improve hazard assessment for future eruptions by better understanding flow emplacement processes and how to use flow models to accurately represent these processes.
-
In 2020, the first of now five eruptions with Halemaʻumaʻu crater at the summit of Kīlauea began while I was at HVO. During this eruption, I participated in helicopter overflights of Halemaʻumaʻu to collect photos for the generation of topographic data of the erupting lava. I am leading a detailed photogrammetric analysis using this data to study the growth of the new lava lake at Kīlauea during 2020-present.
With collaborators at the University of Arizona and the University of Iceland, I helped collect a large UAS image and video dataset during the 2021, 2022, and 2023 eruptions at Fagradalsfjall, Iceland. Using this data, I am initiating a project that will investigate the role of dynamic topography due to emplaced lava on lava flow hazards and flow-field development. Implications of this study include improved understanding of how lava flows can control their own emplacement path and interpretation of inactive lava flow fields and eruptive histories.
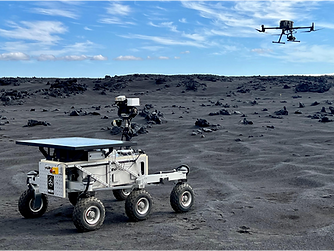
Above: The MESR Rover and a UAS off to explore the Holuhraun lava flow field during the 2022 field campaign in Iceland.
Below: The Gruithuisen Domes on the Moon. Image (c) ASU/NASA/LROC
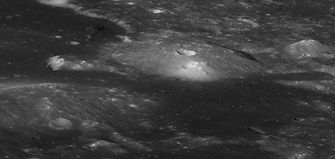
Planetary Volcanism
Volcanism is one of the more ubiquitous surface processes on rocky planets and moons throughout the solar system. I am applying my expertise in terrestrial eruptive processes and the application of UAS to observe volcanic terrain on earth to help better understand and explore volcanic deposits on other planets.
In 2022, I was the UAS operations lead during the RAVEN field campaign at Holuhraun, Iceland. This NASA PSTAR project aims to investigate how a UAS and Rover can best work together to explore planetary surfaces and inform future NASA missions. For the 2022 mission, we simulated a UAS-only mission to develop strategies for using a UAS as a planetary exploration vehicle. Our mission demonstrates how useful a UAS can be for exploring large areas and rough terrain, but also showed limitations when attempting to investigate targets in high detail at outcrop-scale.
While ubiquitous on earth, silicic volcanism on other planets is relatively rare and the formation and eruption of silicic magmas on these bodies is poorly understood. The Gruithuisen Domes on the Moon are a theorized site of lunar silicic volcanism and the location of a future landed robotic mission. I am part of project that is use the Wildcat Hills of Utah as a terrestrial analog for the Gruithuisen Domes. I am leading the task of mapping and characterizing the textures, morphologies, and emplacement history of the silicic lavas of the Wildcat Hills. Our results will help build hypotheses and provide context for the interpretion of observations during the future robotic mission.
